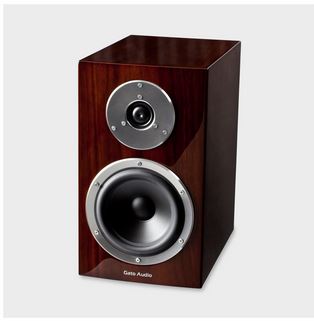
This article follows thestudy of the electroacoustic speaker. We will see here what happens to its properties and its radiation when it is integrated into a cabinet to make a loudspeaker.
Resonance of a driver mounted in a cabinet
Pressure variations in the cabinet
As we have seen, the opposite phase emission from the rear side of the membrane creates harmful destructive interferences, especially in the low frequency range. The integration of the loudspeaker in a cabinet aims to avoid this acoustic short-circuit: the rear radiation is in fact suppressed.

Let V_0 the volume of the box, enclosing air at atmospheric pressure P_0. The volume of air contained in the sealed cabinet varies with the displacement of loudspeaker membrane of surface S when the elongation of the membrane is x, the air volume is V=V_0 + \Delta V with \Delta V = S \cdot x. The air undergoes a succession of compressions and expansions during the vibrations of the membrane.
If we assume, as usual, that these transformations are adiabatic, it follows from Laplace's law that:
\displaystyle{ \frac{ \text d}{\text d V} \left( P\cdot V^\gamma \right) = 0 \iff \frac{ \text{d}P}{\text{d}V} = - \frac{\gamma P}{V} }
Hence, if the volume \Delta V swept by the membrane remains small compared to the total volume of the chamber V_0the resulting overpressure can be written as :
\displaystyle{ p = - \frac{\gamma P_0}{V_0} \Delta V = - \frac{\gamma P_0}{V_0} S x }
Mechanical equation
Let's follow the application of Newton's second law to the loudspeaker seen previously, we obtained the mechanical equation of the speaker:
(m + 2m_\text{R}) \cdot \ddot{x} + (\alpha + 2R_\text{R}) \cdot \dot{x} + k \cdot x = Bl \cdot i
Two changes must be made in the configuration of the closed enclosure:
- On the one hand, since the back radiation has been removed, the factor 2 in front of the radiation resistance R_\text{R} disappears.
- One the other hand, the overpressure p of air enclosed in the cabinet adds a force F whose expression is, according to the result of the previous paragraph : F = p\cdot S = - \frac{\gamma P_0 S^2}{V_0} x
This is a recall force F = - k_\text{a} \, x, with k_\text{a} = \frac{\gamma P_0 S^2}{V_0} the stiffness of the volume of air enclosed in the box.
Assuming that the surface area of the diaphragm is small compared to the surface area of the front panel of the housing, the mass of air displaced at the back of the diaphragm is approximately equal to that displaced at the front. The total mass of the moving assembly will therefore remains m+2m_R
The mechanical equation of the loudspeaker mounted in a cabinet is therefore :
\displaystyle{ \boxed{ (m + 2m_\text{R}) \cdot \ddot{x} + (\alpha + R_\text{R}) \cdot \dot{x} + \left( k + k_\text{a} \right) \cdot x = Bl \cdot i }}
The behavior of the loudspeaker mounted in a closed box can therefore be deduced from that of the loudspeaker mounted on an infinite plane baffle by the substitutions :
- 2R_\text R \rightarrow R_\text R
- k \rightarrow k_\text{b} = k + k_\text a with k_\text a = \frac{\gamma P_0 S^2}{V_0}
We will use the index b for all quantities related to the speaker mounted in a box.
Resonance frequency
Following the approach we used for the speaker mounted on a plane baffle, we end up with the equivalent electrical diagram of the speaker mounted in a box:

The motional resistance and inductance are unchanged, but the motional capacitance undergoes a change:
\displaystyle{\left\{ \begin{array}{ll} L_{\text{m,b}} = \frac{m +2m_\text{R}}{(Bl)^2}\\ R_{\text{m,b}} = \frac{(Bl)^2}{\alpha} \\ C_{\text{m,b}} = \frac{(Bl)^2}{k_\text b} \end{array}\right.}It follows that the resonance of the motional circuit R,L,C in parallel will occur at the pulsation :
\displaystyle{\left( \frac{\text{d}\underline{Z}_\text{mot}}{\text{d}\omega}\right)_{\omega=\omega_b} = 0 \iff \omega_b = \frac{1}{\sqrt{L_{\text{m,b}} \cdot C_{\text{m,b}}}} = \sqrt{\frac{k_b}{m + 2m_\text{R}}}}The numerator of the expression is larger than in the case of a flat baffle mounting, and the denominator is the same: the resonance frequency of the loudspeaker is therefore increased when it is installed in a cabinet.
The speaker mounted in a cabinet will therefore have a higher bass cut-off frequency.
The loss of emission in the bass will be all the more marked as the stiffness k_\text a of the air contained in the chamber will be important, this one increasing when the volume V_0 of the cabinet decreases. The crossover frequency of the speaker will be multiplied by \sqrt{2} when k_\text a = k. This threshold gives the order of magnitude of the minimum volume of the box to be used.
It is customary to introduce here the air volume equivalent to the suspension V_\text{as}, usually published in the loudspeaker manufacturer's data. The stiffness k of the loudspeaker would be obtained with a volume of air
\boxed{ \displaystyle{ V_\text{as} = \frac{\gamma P_0 S^2}{k} }}
To mount a loudspeaker in a closed cabinet without increasing the crossover frequency too much, you will need a cabinet volume at least a few times greater than V_\text{as}.
Example
Let's illustrate these words with a numerical example. Let's recall the manufacturer's data of the Monacor SP-165PA loudspeaker that we took as an example in the previous article: :
- Effective membrane area S = 137\times10^{-4}\,\text{m}^2
- Mass of the moving part (coil + membrane) m = 8,2\times10^{-3} \,\text{kg})
- Stiffness of the elastic suspension k = 4,0\times 10^{3} \,\text{N}\,\text{m}^{-1})
- Mechanical resistance of the suspension \alpha= 1,2 \,\text{kg}\,\text{s}^{-1}
- Coil's resistance R_e = 6.9\,\Omega
- Coil's inductance L_e= 0,50 \times10^{-3} \,\text{H}
- Force factor Bl = 7,1 \,\text{T}\,\text{m}
We have for this speaker \displaystyle{ V_\text{as} = 6,6 \,\text{L} }. If one chooses to mount it in a volume box V_0=40\,\text{L} \approx 6 V_\text{as}we will have : k_\text a = \frac{\gamma P_0 S^2}{V_0} = 6,7 \times 10^2 \,\text{N}\,\text{m}^{-1}
The total stiffness will be: k_\text b = k + k_\text a = 4,7 \times 10^3 \,\text{N}\,\text{m}^{-1}
And the resonance pulsation: \omega_\text b = \sqrt{\frac{k_b}{m + m_\text{R}}} = 654 \, \text{rad} \, \text{s}^{-1} = 1,08 \, \omega_0
The cutoff frequency will have increased by 8%, from 96 to 104 Hz.
Acoustic radiation
Transfer function
The equivalent acoustic diagram for the speaker mounted in a cabinet is :

- Excitatory pressure \underline{p}_g=\underline{P}_g \exp (j\omega t) with \underline{P}_g = \frac{(Bl)\cdot\underline{U}}{S\cdot R_e}, in units of Pascals.
- Acoustic flow \underline{q} = \underline{Q} \exp (j\omega t), in \text{m}^3/\text{s}
- Acoustic resistances, in units of \text{Pa}\cdot\text{s}/\text{m}^3:
- R_{ae}=\frac{(Bl)^2}{S^2 R_e} (electrical losses in the coil)
- R_{ab}=\frac{\alpha}{S^2} (frictional losses)
- Acoustic compliance, in units of \text{m}^3/\text{Pa} : C_{ab}=\frac{S^2}{k_b}.
- Acoustic mass, in units of \text{kg}/\text{m}^4 : M_{ab}=\frac{m + m_\text{R}}{S^2}
- Acoustic resistance of front radiation, R_{ar}=\frac{R_\text{R}}{S^2}
Let's call:
- \delta = \frac{\omega_b}{\omega_s} = 1,08 ;
- Q_{eb} =\frac{1}{\omega_b C_{ab}R_{ae}} the electrical quality factor ;
- Q_{mb} =\frac{1}{\omega_b C_{ab}R_{ab}} the mechanical quality factor ;
- Q_{tb} the total quality factor such that \frac{1}{Q_{tb}} = \frac{1}{Q_{eb}} + \frac{1}{Q_{mb}} .
Let's take the example of the Monacor already studied, mounted in a 40 L box:
Mounting on a flat baffle | Mounting in a 40 L box |
\omega_s = 606 \, \text{rad}\,\text{s}^{-1} | \omega_b = \delta \omega_s = 654 \, \text{rad}\,\text{s}^{-1} |
Q_\text{es} = 1,1 | Q_{eb}=1,0 |
Q_\text{ms} = 5,0 | Q_\text{mb} = 4,6 |
Q_\text{ts} = 0,91 | Q_\text{tb} = 0,83 |
The quality factors decrease when the loudspeaker is integrated into a cabinet, the smaller the volume of the cabinet.
The expression for the flow, obtained by following the approach outlined in the previous article, is then :
\displaystyle{ \underline{Q}_b = \frac{j\delta \nu}{( j \delta \nu)^2 +\frac{j \delta \nu}{Q_{tb}} + 1} \cdot \omega_b C_{ab} \underline{P}_g = \frac{j\delta \nu}{( j \delta \nu)^2 +\frac{j \delta \nu}{Q_{tb}} + 1} \cdot \frac{ \omega_s C_{as}}{\delta} \underline{P}_g }where \nu = \frac{\omega}{\omega_s} is the reduced pulsation defined with respect to the resonance pulsation of the planar baffle speaker and where the second term has been rewritten according to the values of the planar baffle speaker.
Baffle step
When we studied the acoustic monopoleWe have reported that it models the radiation of a closed enclosure at very low frequencies in a very satisfactory way. Indeed, the wavelength being greater than the dimensions L of the baffle (f < c / L), this one is invisible for the emitted sound waves. The radiation is then isotropic in all space.
Then we studied the model of the piston embedded on an infinite baffle, which accurately models the radiation from a speaker at higher frequencies (f > c / L): the wavelength being smaller than the baffle of the box, the sound waves see it as a large baffle. The radiation is then mainly in the front half space. As long as the wavelength is greater than the radius a of the loudspeaker, this radiation is also mostly non-directional.
In the transfer function obtained in the previous paragraph, it will therefore be necessary to use the monopole model for low frequencies and the baffled piston model for higher frequencies. The transition between these two models is called baffle step, or baffle step transition .
Radiated power in both models
The radiated power is obtained from the transfer function in the same way as we obtained it for the radiation of the speaker mounted on an infinite baffle. The difference between the monopole radiation (low frequency) and that of the baffled piston (high frequency) is the radiation resistance R_R, which is doubled for the baffled piston. We thus obtain for the baffled piston:
\displaystyle{ { \mathcal{P}_{ar,b,\text{HF}} = \frac{\rho_0}{4\pi c} \left( \frac{\omega_s^2 C_{as} \underline{P}_g}{\delta^2} \right) ^2 \left|\underline{H}_{P,b}\right|^2} \hspace{1cm} \text{avec} \hspace{1cm}\underline{H}_{P,b} = \frac{\delta^2\nu^2}{( j\delta\nu)^2 +\frac{j\delta\nu}{Q_{tb}} + 1} }In the monopole case, we get twice as much:
\displaystyle{ { \mathcal{P}_{ar,b,\text{BF}} = \frac{\rho_0}{8\pi c} \left( \frac{\omega_s^2 C_{as} \underline{P}_g}{\delta^2} \right) ^2 \left|\underline{H}_{P,b}\right|^2}} = \frac{ \mathcal{P}_{ar,b,\text{HF}}}{2}In the low frequencies, the maximum value of the radiated power is thus divided by 2\delta^4 compared to the same loudspeaker mounted in a flat baffle. In order to optimize the bass cutoff frequency and the sound level, it is therefore in the best interest of the speaker to place it in a cabinet with a sufficiently large volume so that \delta close to 1.
The efficiency \eta_b of the loudspeaker mounted in a closed cabinet is calculated in the high frequencies, where \left|\underline{H}_{P,b}\right| \approx 1 : we thus have \eta_b = \frac{\eta_0}{\delta^4}. In our example, we get 0,6\%.
The sensitivity L_{1,b} of the enclosure is reduced by 10\cdot \log{\delta^4}, i.e. a drop of 1,3\,\text{dB} for the considered enclosure: we obtain L_1 = 90,2\,\text{dB}.
Baffle step transition
At high frequencies, the sound waves are emitted in the front half of the cabinet. At a distance r from the speaker, the emitted power is diluted on a half-sphere of surface 2\pi r^2 and the sound intensity is:
\displaystyle{ I_\text{HF} = \frac{ \mathcal{P}_{ar,b,\text{HF}} }{2\pi r^2}}At low frequencies, sound waves are emitted in all space, the surface on which the energy is diluted is therefore 4\pi r^2. Then we have :
\displaystyle{ I_\text{BF} = \frac{ \mathcal{P}_{ar,b,\text{BF}} }{4\pi r^2} = \frac{I_\text{HF}}{4}}The transition linked to the baffle step is therefore accompanied by a decrease of 6\text{dB} in the bass.
On which frequencies does the baffle step spread?

The diffraction is quantified by the angle \theta of the wave spreading. For the obstacle of dimension L that is the cabinet of the speaker, we have :
\theta = \frac{\lambda}{L}
A wave emitted by the loudspeaker along the baffle will be significantly diffracted to the rear when \theta \approx \frac{\pi}{4}. This corresponds to \lambda_1 \approx 0,7L. The radiation will become isotropic in space when \theta \approx 2\pi, that is \lambda_2 \approx 6 L. The transition frequency is the one for which the attenuation is half of its maximum value, i.e. :
f_\text{step} = \sqrt{f_1 f_2} = \sqrt{\frac{c^2}{\lambda_1\lambda_2} } \approx \frac{1}{2} \frac{c}{L}
f_1 \approx 3 \, f_\text{step}, f_2 \approx \frac{f_\text{step}}{3}
Or
\lambda_\text{step} = \frac{c}{f_\text{step}} \approx 2L
\lambda_1 \approx \frac{\lambda_\text{step}}{3}, \lambda_2 \approx 3 \, \lambda_\text{step}
A good mathematical model of the transition can be constructed using a well-known approximation of the Heavyside step function :
H(f) \approx 6 \times \left( \left( 1 + \exp{ \left( -2 k \ln{\frac{f}{f_\text{step}}} \right) } \right)^{-1} -1 \right)
with k=2 allowing the spreading of the phenomenon over the interval \left[ \frac{f_\text{step}}{3} ; 3 \, f_\text{step} \right] :

Sound level
We can now calculate the sound intensity at high frequencies with the expression obtained above, deduce the sound level and take into account the transition to monopole radiation at low frequencies by adding the baffle step transition.
For our 40L loudspeaker, let's choose a depth of the cabinet of 25 cm and a circular baffle of 22,5 cm of radius, the loudspeaker being mounted in the center of it. I don't recommend such a geometry, but it allows to have only one dimension L=0,45 \,\text{m} to consider. We obtain, with a baffle phenomenon at 380 Hz ranging from 127 Hz to 1.14 kHz :

The green vertical lines indicate the cut-off frequencies at -3dB and the red ones the limits of the baffle step transition
The high-frequency cutoff at 5500 Hz was added artificially from the experimental observations in the previous paper.
It should be noted, however, that in a real case of listening in a room, a significant fraction of the bass sound waves emitted towards the back of the speaker will be reflected by the walls, thus increasing the perceived level of the bass. The baffle phenomenon will therefore cause a decrease of 2 to 4 dB rather than 6 dB.
Note also that, when the box is rectangular, the transition will be smoothed out due to different transition frequencies for the different sizes of the obstacle seen by the wave :


For example, for the same box volume of 40L and the same depth of 25cm, a rectangular baffle of dimension 30 \times 50 \,\text{cm} will have a transition of baffle phenomenon that will extend from \lambda_1 \approx 0,7 \,L_{min}=21\,\text{cm} at \lambda_{max} \approx 6 \,L_{max}=3,0 \,\text{m}, that is:
f_{min}=114\,\text{Hz} \,\, ; \,\, f_{max}=1,63\,\text{kHz}, \,\,f_\text{step}=\sqrt{f_{min}f_{max}}=430\,\text{Hz}

Diffraction from the edges
The problem
In this section, we are interested in the "high-frequency" side of the baffle step: the sound waves are supposed to propagate in a half-space delimited by the baffle.
Let us consider a spherical wave. The Huygens-Fresnel principle states that each point of the medium perturbed by the wave becomes itself a secondary elementary source, the combination of the perturbations generated by these secondary sources constituting the new wave front:

When the wave propagating along the baffle reaches its periphery, it encounters a discontinuity since the volume available for its propagation changes: it passes from a half-sphere to a sphere. The pressure drops by a factor of 2 and the secondary sources no longer form a coherent whole with their predecessors, they must be considered as a new source. We speak of diffraction by the edge of the baffle.
Due to their phase shift, resulting from the delay induced by the propagation to the edge of the baffle, the direct wave and the diffracted wave interfere at the point of observation: the sound pressure will show peaks when the waves are in phase and troughs when they are in phase opposition.
Case of a circular baffle
In order to simplify and focus on the physical effects, we will first restrict ourselves to the unfavorable case of a circular baffle observed on axis.
We are interested here in high frequencies: let us thus change our loudspeaker of study for a tweeter Dayton DC28FT of radius a=14 \,\text{mm}, of resonance frequency f_s = 834 \,\text{Hz} and of total quality factor Q_\text{ts} = 0,50. This loudspeaker is mounted in a closed enclosure, in the center of a circular baffle of radius R= 22,5 \,\text{cm}. There is no modification of the resonance frequency for the tweeter: it is factory mounted in an airtight box (the box would serve, in a real case of two-way speaker, to remove the acoustic short circuit of the bass speaker).
Its theoretical response curve is then, taking into account the baffle step:

The distance covered by the wave when reaching the edge of the baffle is equal to the radius R of the baffle. The edge of the baffle thus behaves like a second source emitting at the same frequency as the loudspeaker but with a delay \tau = R/c, the time it takes to reach the edge of the baffle from its center.

The direct wave propagating in a half-space is at point M :
\displaystyle{ p_0 = \frac{j \omega \rho Q_0}{2\pi} \cdot \frac{\exp{j \left( \omega t - k r_0\right) }} {r_0} }
The diffracted wave emitted by an infinitesimal secondary source of length \text{d}\mathcal{l} = R\cdot \text{d}\varphi is written as follows:
\displaystyle{ \text{d}p_1 = D(0) \cdot \frac{j \omega \rho Q_0}{4\pi} \cdot \frac{ \exp{ \left[ j \left( \omega t - k \left(r_1+R\right) \right)\right]} } {r_1 + R} \cdot \frac{\text{d}\varphi}{2\pi} }
where D(0) is the directivity factor of the speaker along the baffle.
In far field, we have r_1 \approx r_0 and the difference in amplitude due to the additional distance traveled R is negligible. Therefore:
\displaystyle{ \text{d}p_1 = D(0) \cdot \frac{1}{2} \cdot p_0 \cdot \exp{\left( -j k R \right)}\frac{\text{d}\varphi}{2\pi} }
The integration along the circle is trivial, finally:
\displaystyle{ p_1 = D(0) \cdot \frac{1}{2} \cdot p_0 \cdot \exp{\left( -j k R \right)} }
The resulting pressure at the observation point is therefore :
\displaystyle{ p = p_0 + p_1 = p_0 \left( 1 + D(0) \cdot \frac{1}{2} \exp{ \left(- jkR \right) } \right) }
Direct and diffracted waves interfere at the observation point via the term in parentheses; the effective sound pressure is :
\displaystyle{ p_\text{eff} = \frac{ \left| p \right| }{\sqrt{2}} = p_{0,\text{eff}} \left( 1 + D(0) \cdot \frac{1}{2} \cos{ \left(kR\right) }\right) }
The interference will be constructive when the direct and diffracted waves are in phase at the observation point, i.e. when k R = m \cdot2\pi with m a positive integer, that is \lambda = R/m or f = m c /R. The waves will be in phase opposition, generating destructive interference, when k R = \left( m + \frac{1}{2} \right) \cdot2\pi, that is \lambda = R/\left( m + \frac{1}{2} \right) or f = \left( m + \frac{1}{2}\right) c /R.
The speaker becomes very directional at high frequencies (ka >= 4the phenomenon fades away:

The sound level variations are therefore of the order of 10 dB maximum (the pressure varies by a factor of 3 between 0.5 and 1.5, the sound intensity varies by a factor of 9 and 10 \times \log{9} = 9,5.
We can now compute the sound intensity I=\frac{p_\text{eff}^2}{\rho c} and the resulting sound level:

The first trough, corresponding to \lambda / 2 = R, is partially hidden by the baffle step (the wavelength is long, the radiation of the speaker is therefore almost on a sphere and there is little diffraction). The first bump at the end of the baffle-step transition corresponds to \lambda = R then the next trough at \lambda = \frac{2}{3}R, etc.
Let us observe an enlargement of the area of interest, in linear frequency scale:

The peaks are spaced at about 1500 Hz, the corresponding wavelength is \lambda = \frac{c}{f} = 22,8\,\text{cm} : we can thus measure the radius of the baffle.
Note here that the amplitude of the variations will be reduced by rounded edges.
Off-axis observation
We now place ourselves off-axis, in a direction defined by the angle \eta relative to the axis of symmetry of the baffle:

A little bit of geometry allows us to see that the additional path difference of the diffracted wave relative to the direct wave is \delta = R \sin{\eta}\cos{\varphi}, the corresponding phase shift is \phi_0 = k\delta.
The direct wave has therefore the expression :
\displaystyle{ p_0 = D\left(\frac{\pi}{2}-\eta\right) \cdot \frac{j \omega \rho Q_0}{2\pi} \cdot \frac{\exp{j \left( \omega t - k r_0\right) }} {r_0} }And we will have at the observation point, for an infinitesimal secondary source \text{d}p_1 :
\displaystyle{ \text{d}p_1 = D(0) \cdot \frac{1}{2} \cdot p_0 \cdot \exp{\left( -j k (R - \delta) \right)}\frac{\text{d}\varphi}{2\pi} }The wave diffracted by the edge of the baffle is thus :
\displaystyle{ p_1 = D(0) \cdot \frac{1}{2} \cdot p_0 \cdot \exp{\left( -j k R\right)} \cdot \int_0^{2\pi} \exp{\left( j k R\sin{\eta}\cos{\varphi}) \right)} \frac{\text{d}\varphi}{2\pi} }The integral in the last term is : J_0\left(k R \sin(\eta)\right), Bessel function of order 0 :
\displaystyle{ p_1 = D(0) \cdot \frac{1}{2} \cdot p_0 \cdot \exp{\left( -j k R\right)} \cdot J_0\left(k R \sin(\eta)\right) }
Summing the direct wave p_0 (affected by the directivity factor D(\frac{\pi}{2}-\eta) ) and the diffracted wave p_1, then calculating the corresponding sound intensity and deducing the sound level in the same way as before, we obtain the following curves:

It is not surprising to see that the diffraction by the edges is smaller off-axis: indeed, the various secondary sources around the baffle are no longer all in phase. This calculation shows that when we try to correct the peaks and troughs on-axis by digital filtering, we generate peaks and troughs off-axis.
Rectangular baffle
Let's take a look at the more concrete case of the rectangular baffle, observed on axis. Its geometry is summarized in the following figure:

We have kept the dimensions of the box used in the calculation of the baffle phenomenon: L_1=50\,\text{cm}, L_2=30\,\text{cm}. The tweeter is located at the coordinates x_t = y_t = 20\,\text{cm}.
The expression of the direct wave is identical to that obtained in the case of the circular baffle, and an infinitesimal source located at the coordinate \varphi will generate at the observation point, with the same simplifications as before:
\displaystyle{ \text{d}p_1 = D(0) \cdot \frac{1}{2} \cdot p_0 \cdot \exp{\left( -j k b(\varphi) \right)}\frac{\text{d}\varphi}{2\pi} }
The integration is done numerically, by pieces for each of the edges of the baffle. For example, for the upper edge, we have :
\displaystyle{ p_{1,\text{top}} = D(0) \cdot \frac{1}{2} \cdot p_0 \cdot \int_{\arctan{\frac{L_1-y_t}{L_2-x_t}}}^{\frac{\pi}{2}+\arctan{\frac{x_t}{L_1-y_t}}} {\exp{\left( -j k \frac{L_1-y_t}{\sin{\varphi}} \right)}\frac{\text{d}\varphi}{2\pi} } }
The interference term has the shape given below.

We deduce the sound level of the loudspeaker mounted in the cabinet as above:

As expected, the effects of diffraction by the edges of the baffle are much less pronounced than with a circular baffle (the secondary sources are never all in phase with the direct wave). Here, as with the circular baffle, the measurement of the frequency differences of the different peaks allows us to deduce the distance between the loudspeaker and the edges of the baffle. For example, the two most visible peaks on the graph are 3400 Hz apart, which corresponds to a wavelength of 10 cm, the distance between the loudspeaker and the top of the cabinet.
The directivity study is also performed by numerical integration, adding the additional step difference as in the case of the circular baffle \delta = b(\varphi)\cdot\sin{\eta}\cdot\cos{\varphi} :

Conclusion
We have seen in this article the consequences on the acoustic radiation of the sealing of a loudspeaker in a box: increase of the resonance frequency, baffle phenomenon and diffraction by the edges of the baffle.
A possible way to counter the increase of the resonance frequency and thus of the low cutoff of the loudspeaker is to integrate a vent in the cabinet to realize a bass-reflex speaker. The next article will be dedicated to this system.
Sources
Francis BROUCHIER – Haut-parleurs et enceintes acoustiques : Théorie et pratique
Jean Fourcade – Utilitaires Scilab pour le calcul et l’optimisation d’enceintes bass-reflex